🔋 Unleashing a Battalion of Batteries #017
getting schooled on batteries with Nicholas Yiu of Intercalation Station
TLDR: Batteries are a critical energy storage system which close the gap between energy consumption and energy generation peaks. Key applications are in EVs, renewables, consumer electronics, and military. Software plays a key role in this physical hardware. The minerals supply chain is complex with geopolitical and ethical concerns. A shortage is likely. It’ll be a tough race.
We live in an era of abundance. Not to dismiss the importance of solving food insecurity, but most of us today no longer need to worry about our next meal. We have more than what we need. Fortunately, with the modern marvels of supply chain and refrigeration, I don’t have to walk to a fruit tree or pull out my bow and arrow and scan the horizon for wild game every time I get hungry. I simply walk to the kitchen. If I’ve been on top of my game, then I will thank the past me for stocking the fridge and pantry. Thanks to food preservation methods like fermentation, refrigeration, and drying, we’re able to extend the shelf life of food AKA energy for humans. It’s nice to have forms of human energy storage like a pantry and refrigerator because my demand peaks (when my tummy rumbles) rarely ever matches when and where the supply (food) is created.
Unfortunately, that’s not how the grid operates. Both shortages and surpluses can lead to blackouts. The grid must constantly balance the demand and supply of electricity. Energy consumption and energy generation curves don’t perfectly match, just like my hunger fluctuations and when food is readily accessible. Peak times for energy consumption tend to be when we’re at home: getting ready in the morning and having dinner with the fam in the evening. Once the supply side, production happens whenever the sun is shining and the wind is blowing. If you overlay the energy consumption and generation curves on top of each other, you get a duck:
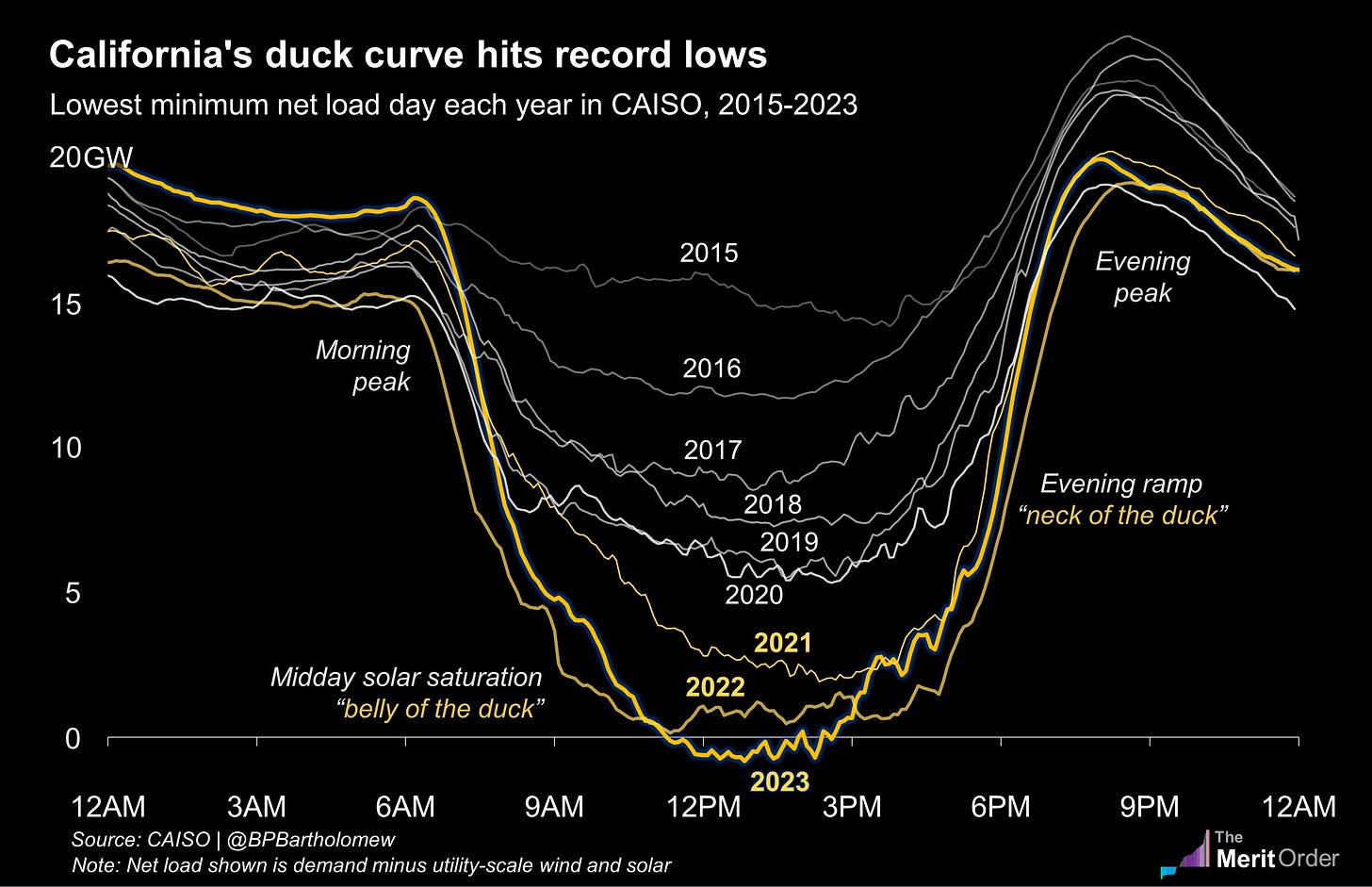
Due to the inherent constraint of always keeping the flow of electricity in homeostasis, whenever there’s more power being generated than consumed, the utility company will need to figure out a way to reduce production, which often results in temporarily shutting off parts of the grid. During periods of curtailment, super expensive utility-scale renewable infrastructure sits idle, a complete waste of resources.
The good news is that there actually are ways we can store energy. And just like the multiple forms of food storage (refrigerate, ferment, dry), there are different forms of energy storage. Thermal stores energy in the form of heat and then converts it to electricity whenever needed. Mechanical uses gravity (pumped hydro) or tight spaces (compressed air) to store energy for later. And then there’s electrical - the one we’ll be focusing on today.
Batteries, the electrical type of energy storage, are compelling for many reasons, but especially because they’re a flexible, adaptive technology (not everyone has a hill for pumped hydro) that continues to exponentially decrease in cost just like other climate-relevant technologies (solar, DNA sequencing, growing meat in a lab).
To further understand the role of batteries in the transition to a decarbonized economy, I called up Nicholas Yiu, battery buff and co-founder of Intercalation Station, the best* resource on batteries out there (*according to me). Our conversation starts out broad with the basics so that we can build up from first principles. From there, we go on to discuss:
Battery Type Breakdown
Use Cases for Batteries
Batteries for EVs
Renewables and Storage Duration
Minerals and Supply Chain
Software for Batteries
Future Predictions
The Current State of Batteries
Nicholas: Lithium ion batteries have been around since the 70s and they've only recently become popular from a commercialization standpoint because of the downwards trending cost. Today, they're more or less about a hundred dollars per kilowatt hour (unit of energy for batteries).
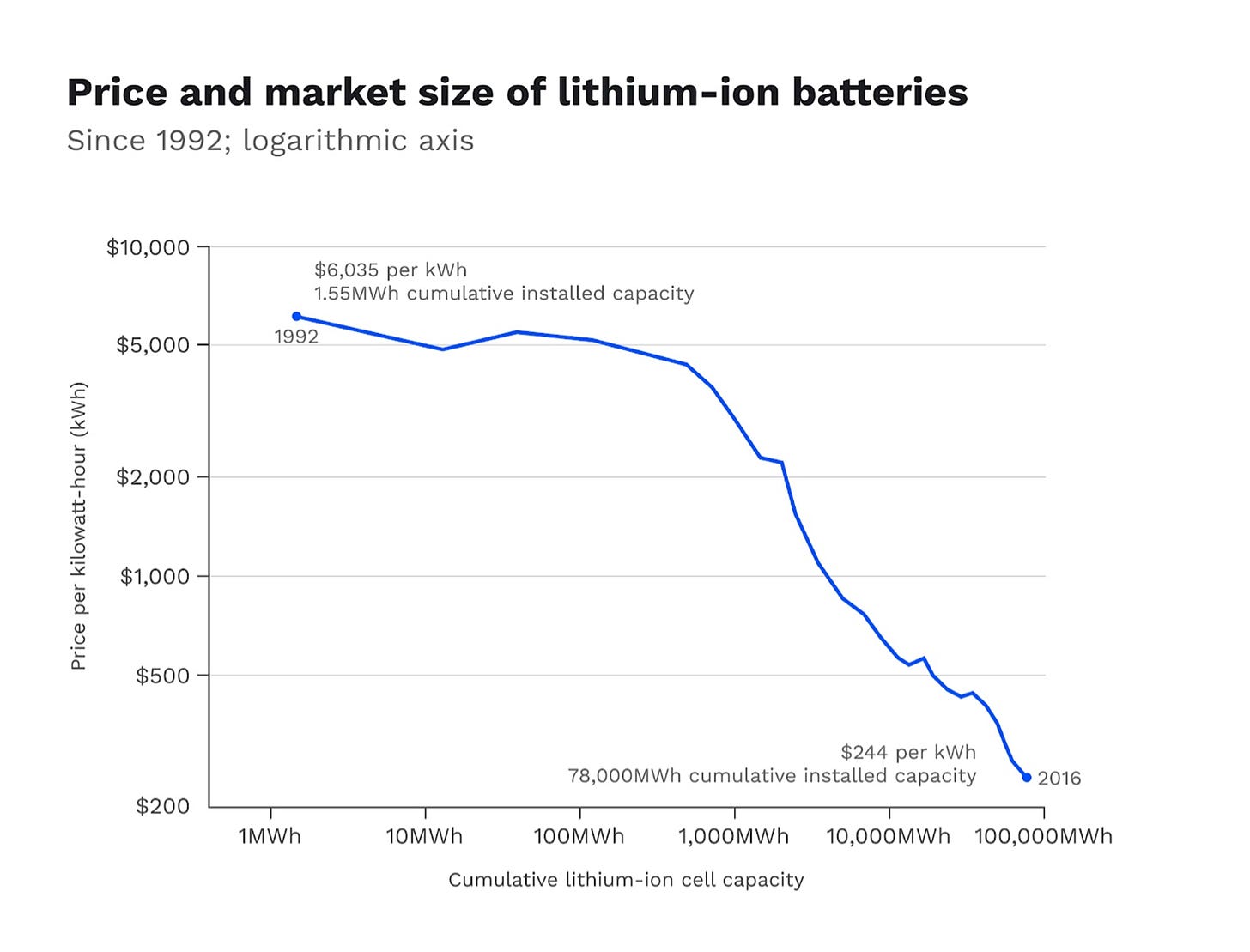
When you translate that to a car battery pack, it would've cost over $50,000 in 2013. There wasn’t much interest in 2015 and it wasn’t until 2019 onwards when costs started dropping due to a higher volume of Asian production and new manufacturing technologies coming online to produce better batteries faster. It’s now much more affordable, generally between $100 to $200 per kWh (and even between $50-100 per kWh at some sites) and that's why they’ve become so popular over the last few years for all applications like mobility and stationary storage.
Right now the most advanced commercially available energy storage system is based on lithium ion batteries. They’re crucial for renewable energy storage, mobility applications, and other applications. There are a lot of areas that can be electrified via batteries and displace fossil fuel usage.
Battery Type Breakdown
Nicholas: When we're talking about rechargeable batteries, the lithium ion battery is the most commercially dominant battery out there. There are several components inside the battery but the main idea is that it's an electrochemical sandwich. You have the current collector, which is a thin sheet of metal to help conduct electrons. Then there are battery electrode materials - these are the active materials that actually hold the lithium. The electrolyte is sort of the jelly in between your electrodes that allow the lithium ions to move between electrodes. This is all connected via an external electrical circuit to power the load.
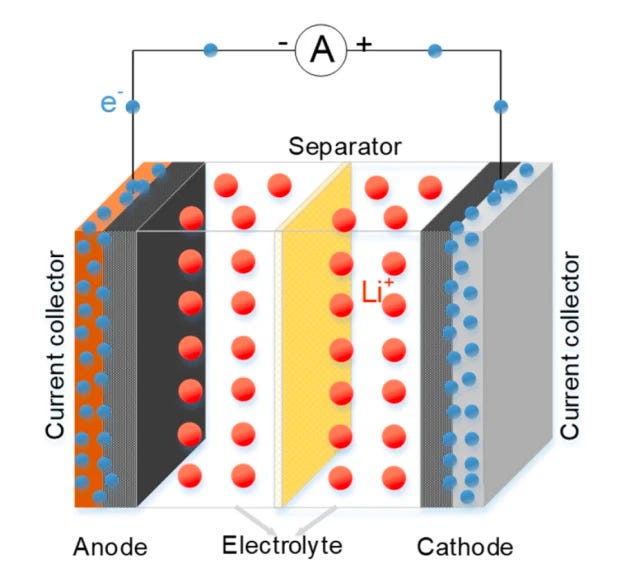
Looking at other non-lithium based technologies, there are different sorts of alternate chemistries. The main one is sodium ion which is popular these days - basically replacing your lithium ions with sodium ions. The upside is that sodium is widely available and doesn’t rely on as many critical metals as lithium ion batteries. In addition, the performance of sodium ion cells can be competitive with lithium ion in certain applications. The downside of sodium ion is it's a bit bigger which means it doesn't hold as much energy per mass. It also could mean that it's slower in diffusion, so it doesn't charge or discharge as quickly. Not ideal for high power situations where you might need to use a big burst of energy when you're taking off and landing, for example, in an electric helicopter. Lithium and sodium are the two main chemistries right now. There are a few more in the research infancy, for example, aluminium or magnesium which are also in early commercialization days.
Use Cases for Batteries
Nicholas: The main applications that everyone is really excited about are largely investor driven. This means technologies with massive markets. Sometimes, they will be really excited about things that are really flashy as opposed to what makes the most sense from a climate perspective.
The first and foremost is mobility which includes electric vehicles, scooters, and electric vehicle takeoff and landing (eVTOLs). There’s some exploration into maritime; so some electric boats that can cross short distances, as well as an exploration into electric planes. The downside for these applications is that batteries are always going to be very comparably heavy and not as energy-dense as kerosene. It’s going to be difficult to electrify things like commercial aeroplanes or larger boats where weight is a determining factor. For vehicles like cars and trucks, the battery size is reasonable for these applications compared to what you would need for a plane. Typical sizes for that are 30 kilowatt hours for a Prius, all the way up to several hundred kilowatt hours for commercial trucks and buses. Hummers are excessive and just way too big for just one vehicle. No one needs a battery that big.
When it comes to using lithium ion batteries for grid storage applications, the diversity in designs and capacities can be as varied as those of fossil fuel plants. These storage systems can be paired with renewable energy generation to help store the energy generated from solar plants and wind farms. They can also deliver meaningful financial returns by doubling as energy trading machines, doing various forms of energy arbitrage where power is bought when grid prices are cheapest and sold when grid prices are highest. In terms of scale, these renewable energy storage systems are similar scale to hundreds of vehicle batteries combined. Grid storage for renewable energy storage and batteries for electric mobility are the two main applications with the biggest markets where you can get the most impact on the environment.
There are also smaller applications like consumer electronics: smartphones, laptops, smartwatches, etc. This is also a big market, but I find it's often used as a stepping stone to get to the bigger markets. For example, a company will develop a new chemistry and prove that it works in a small system like a smartwatch. [ex: Sila Nanotechnologies working with Whoop] The batteries can be the size of a coin and it’s a good way to demonstrate that your battery chemistry works in a small system. The next step is to put it in cars. The development type process to go from watch-size to car-size is really expensive so it’s good to start small, especially with new processes and new chemistries, and fine-tune your manufacturing.
In terms of novel tech that is very expensive (and likely wouldn’t have a buyer in the average market), a lot of battery companies turn to military applications because there is no price sensitivity. They just want smaller, better and highest-performing batteries, and they don't care very much about the price. I would bucket those (mobility, energy storage, consumer electronics, military) as the main four branches of battery applications.
Electric Vehicles (EV weight, range anxiety, charging experience)
Nicholas: I do spend a lot of time on the fast-charging side of things. First of all, there are some very good studies led by London battery consultancies Exawatt and Minviro. You don't even need studies to be honest. I can look at my own daily life patterns.
Say I do a long road trip where I might be thinking actively about where can I charge my car after four hours of driving. Where do I take a break? Is this a good time to charge? Will there be enough chargers? Will my car make it to the charging station? That might happen to me once a year. Otherwise, I'm just driving to the tube station in London which is 10 minutes away, or just doing groceries which is a five-minute drive. This links back to the white paper that 80% of trips are under 10 miles for the average UK driver, but I suspect similar habits in many places around the world.
But at the same time, many consumers have this slightly irrational fear that this is just the way cars need to be and they always want to be able to “fill up” in three minutes and drive until the gas runs out and then just top up again at the nearest gas station. A switch in driver mindset needs to occur with customer education because fast charging like that is going to wreck your batteries pretty quickly.
There are a lot of new chemistries coming out that will help facilitate fast charging with different materials and longer lifetimes. Niobium anodes are a well-known material that fast charges quickly [example companies: Echion, Nyobolt]. A lot of the silicon anode tech [Sila Nano, Nexeon] can fast charge quickly and lithium metal anodes too [SES, Cuberg]. These are all things in various levels of commercialisation and will be fun to watch this decade. There’ll be new charging infrastructure as well, including better software for your battery management systems and different types of charging station hardware. For example, there are a handful of wireless charging companies coming to fruition. Tesla recently acquired Wiferion, a German wireless charging startup, for $76 million. I currently consult for a London-based startup called Gaussion, working on using magnetic fields to reduce ionic resistance and enable fast charging with minimal battery degradation. But we will definitely need more customer education so they realize that it's okay to stop once in a while and charge at home overnight, and it won’t be the same as refuelling with fossil fuels (and that’s okay).
Renewables and Storage Duration
Nicholas: Storage duration is how long the energy in your system can last. For a typical lithium ion system, if you are using your car at 0.5 C rate [C-rate is the rate at which a battery is charged or discharged relative to its capacity], you can use it for two hours. That’s the typical kind of storage duration that you can expect for vehicle batteries. With 10 hours, maybe you'll charge it once a week if you drive an hour-ish per day.
For other systems, that’s not enough. Cities might want energy storage backed up for multiple days or even multiple weeks. That's when you start needing to either expand your lithium-ion storage in that city, which becomes very expensive or you look for larger systems, which can potentially hold a lot more energy for longer and be cheaper. Hydropower, for example, has been around for a long time… It's just water on a hill. That could potentially last for years, and you can extract it when you want, which is helpful and cheap because all you need is water on a dam. Since it's an infrastructure project, it can last for a long time. The downside to that is you need a big hill and a region that has water. If your country just doesn't have hills, then you are struggling with deploying that kind of storage.
So you might look at alternative chemistries and one that comes to mind is iron-based chemistries which are dirt cheap. They're basically rusting and de-rusting and using the reactions from that to drive energy storage. It’s a much cheaper system, and it’s less energy dense therefore the system will be bigger, but for these applications, it doesn't really matter because size isn't the priority here. The priority is storing energy for a long time. I think Form Energy systems can last 100+ hours.
The majority of batteries in vehicles are only used a couple hours a day and so they're just sitting around for the rest of the 24 hours. It's a big waste that they're just sitting there. Companies are starting to think about ways to use lithium ion batteries in homes or in the workplace so you have a virtual network of batteries in vehicles to feed back into the grid to smooth out demand and store energy when you have high output (vehicle-to-grid).
This is a non-exhaustive list of energy storage technologies, and many others could be talked about including mechanical (like gravity storage or compressed air), thermal (molten salt), hydrogen, and others.
Minerals and Supply Chain (geopolitical tensions, ethical dilemmas, shortage?)
Nicholas: There's a shortage declared if demand outpaces supply. It’ll depend on how demand is forecasted by these companies. Forecasts have been wrong in the past, but yes, I do believe that there will be a shortage of critical battery minerals. Even in the case that there isn't a shortage, the question is: Do we want to continue buying a supply of certain materials from certain countries?
Right now, the majority of nickel comes from Indonesia. They will cut down rainforests and displace local populations to mine nickel in those areas. Likewise, the majority of cobalt is mined in the Democratic Republic of the Congo where there's a lot of child labor through artisanal mining practices. While there is enough supply from there, the question is: Do we want to continue mining from those areas to get the cobalt and the nickel to feed into our batteries?
One alternative solution is to use batteries that don't require these materials.
This comes back to the initial conversation. For example, there is potential for sodium ion batteries which are not reliant on those metals. There's potential for lithium Iron phosphate (LFP) batteries. And that's completely not linked to nickel and cobalt at all. It's an iron-based chemistry so it’s more environmentally- and socially-friendly. There are some pros and cons to LFP compared to nickel and cobalt-based batteries and I think ultimately there’ll always be nickel and cobalt-based batteries because they’re higher performing and there will always be non-negligible market demand for higher-performing applications.
Factories right now are producing batteries and competing based on price point, but moving forward, there's going to be a lot more focus on the environmental footprint, where metals come from, and how much carbon is emitted per battery in the factory. The European battery passport has started to implement this where they're requiring a certain amount of battery to come from within Europe if it's a local battery. Similar things will be implemented in the US I believe.
So then the question is if we're not getting cobalt on nickel and other metals from these countries, where do we get it from? I've been spending loads of my free time researching deep sea mining, which I also know is a pretty controversial thing, but is it truly worse than what we're doing right now? This question is open for debate, and I don’t know if we will have an answer in the next few years, and I welcome people to DM me to chat and challenge me about it.
Software's role for batteries (battery life, charging rates)
Nicholas: So many things! I'll start with battery life. The way a battery works from a controls standpoint is you'll apply a current when you want to use (charge or discharge) it. In an EV context, you draw current when you are accelerating (discharging) and then you plug it back into a wall (charging) when it's not in use.
This current needs to be controlled by something inside the car - something like a chip in your battery pack, or maybe it's computed in the cloud. You can measure performance parameters in the battery, like the voltage that’s going up and down between a few preset points of the system. You might also measure the temperature of different areas of the battery pack. Temperature is a big deal because nothing lasts forever and batteries will degrade at a faster rate at higher temperatures.
It’s crucial to understand how your batteries are being operated. Even within the same area in a pack, one cell (an individual component in a battery pack) on the left-hand side might behave differently from one cell on the other side. So having a good software system to measure all the data that's coming from these cells, from voltage to power to temperature can be critical to optimize the lifetime of the battery. Choosing good voltages, knowing which batteries are failing, and catching batteries that will overheat before they do overheat are just some ways that software can be used.
I want to give a shoutout for what I do today - I currently work for a London-based startup called About:Energy which makes digital twins for battery systems. It takes a lot of time, money, and resources to build a battery pack just to see how it’ll perform. We want to cut this problem out. Designers of battery packs can use our software tools to virtually experiment with cells from various manufacturers, for example, a simulated Panasonic or Samsung cell. They can run these virtual cells through simulations to assess their voltage, current, and temperature responses, along with a lot of other physics-based metrics like lithium plating amount or lithium-ion concentration. This allows engineers to determine the most suitable option for their specific needs without having the high costs of building a real-life pack.
One more point when thinking about the end-of-life of the batteries and when you want to take the battery out because it’s no longer fit for purpose… what happens now? Do I put it into recycling or do I put it into a second life? If I put it in a second life, it could be another 10 years in operation. This is another place where software could be handy because you get the entire lifetime data point for the battery. You can basically say, “Based on how this cell was used before, it’s now best for using it in home storage or maybe now it's just unusable for everything because it's just so burned out.” So you might just wanna recycle it at that point.
Future of Batteries / Predictions
Nicholas: The reason I'm always so excited is because it's not just a tech game anymore - it's a geopolitical race. All countries are racing to set up their manufacturing and Asian countries have done a really good job for the last couple decades in manufacturing and they're investing a ton of capital into churning out these batteries. The US and EU are trying to make as many factories as possible.
In terms of how crazy I think things will get, it's probably a bit of a bleaker prediction. Firstly, there are going to be supply shortages of certain materials. However, there's more attention to building factories than figuring out where these materials are gonna come from to feed those factories. It's a possibility that these factories will only be operating at much less than 100% capacity either because they can’t get their materials or they have manufacturing teething problems… Factories are playing catchup with the engineering and manufacturing experience that an Asian manufacturer has.
It’s going to be hard to compete and catch up with Asian manufacturers. What we can do to stay one step ahead (which we've done well in the past) is battery tech innovation. For example, there is currently a lot of innovation in electrode tech for better performance, sodium ion batteries for stationary storage, novel manufacturing techniques to reduce waste, improved software for better system designs, new ways to use batteries altogether with second life, etc. But if it's making standard lithium-ion batteries and trying to compete against Asia, I think that's gonna be difficult in the current iteration. Every factory starting now is at least a handful of years behind the Asian companies.
Look at how well China has done in the past and how quickly they can do things and how strong their workforce is. A lot of factory operations right now in the US have a talent shortage. There are many plans for gigafactories in certain states in the US - now we just need to make sure we can fill those factories with a large number of staff with specific skills and talent. This is easier said than done. It’s going to be a long and hard journey that’s exciting to participate in.
Further Reading on Batteries
Battery Technology Changed the World…and Then It Stalled by Anna-Sofia Lesiv
Contrary Research’s deep dive on Redwood Materials, a battery recycling startup
Looking for more?
Intercalation is currently open to consulting engagements in battery-related projects! Reach out to Nicholas if you’re interested!